[Source:
Oliver Tickell -
Thorium: Not ‘green’, not ‘viable’, and not
likely.]
Numerous advantages for thorium as a nuclear
fuel and for the LFTR design over conventional
solid fuel reactors have been claimed. In this
section we consider each of these claims in
turn.
☣ Abundance of thorium relative to uranium
Claim: Thorium is several times more
abundant in the Earth's crust than uranium.
Response: Thorium (232Th) is
indeed more abundant than uranium, by a factor
of three to four. But whereas 0.7% of uranium
occurs as fissile 235U, none of the thorium is
fissile. The world already possesses an
estimated 1.2 million tonnes of depleted uranium
(mainly 238U), like thorium a fertile but
non-fissile material. So the greater abundance
of thorium than uranium confers no advantage,
other than a very marginal advantage in energy
security to those countries in which it is
abundant.
☣ Relative utility of thorium and uranium as
fuel
Claim: 100% of the thorium is
usable as fuel, in contrast to the low (~0.7%)
proportion of fissile 235U in natural uranium.
Response: Thorium must be
subjected to neutron irradiation to be
transformed into a fissile material suitable for
nuclear fuel (uranium, 233U). The same applies
to the 238U that makes up depleted uranium,
which as already observed, is plentiful. In
theory, 100% of either metal could be bred into
nuclear fuel. However, uranium has a strong head
start, as 0.7% of it is fissile (235U) in its
naturally-occurring form.
☣ Nuclear weapons proliferation
Claim: thorium reactors do not
produce plutonium, and so create little or no
proliferation hazard.
Response: thorium reactors do not
produce plutonium. But an LFTR could (by
including 238U in the fuel) be adapted to
produce plutonium of a high purity well above
normal weapons-grade, presenting a major
proliferation hazard. Beyond that, the main
proliferation hazards arise from:
· the need for fissile material (plutonium or
uranium) to initiate the thorium fuel cycle,
which could be diverted, and
· the production of fissile uranium 233U.
Claim: the fissile uranium (233U)
produced by thorium reactors is not
“weaponisable” owing to the presence of highly
radiotoxic 232U as a contaminant.
Response: 233U was successfully
used in a 1955 bomb test in the Nevada Desert
under the USA's Operation Teapot and so is
clearly weaponisable notwithstanding any 232U
present. Moreover, the continuous
pyro-processing / electro-refining technologies
intrinsic to MSRs / LFTRs could generate streams
of 233U very low in 232U at a purity well above
weapons grade as currently defined.
☣ Safety
Claim: LFTRs are intrinsically safe,
because the reactor operates at low pressure and
is and incapable of melting down.
Response: the design of molten
salt reactors does indeed mitigate against
reactor meltdown and explosion. However, in an
LFTR the main danger has been shifted from the
reactor to the on-site continuous fuel
reprocessing operation – a high temperature
process involving highly hazardous, explosive
and intensely radioactive materials. A further
serious hazard lies in the potential failure of
the materials used for reactor and fuel
containment in a highly corrosive chemical
environment, under intense neutron and other
radiation.
☣ State of technology
Claim: the technology is already
proven.
Response: important elements of
the LFTR technology were proven during the 1970s
Molten Salt Breeder Reactor (MSBR) at Oak Ridge
National Laboratory. However, this was a small
research reactor rated at just 7MW and there are
huge technical and engineering challenges in
scaling up this experimental design to make a
'production' reactor. Specific challenges
include:
· developing materials that can both resist
corrosion by liquid fluoride salts including
diverse fission products, and withstand decades
of intense neutron radiation;
· scaling up fuel reprocessing techniques to
deal safely and reliably with large volumes of
highly radioactive material at very high
temperature;
· keeping radioactive releases from the
reprocessing operation to an acceptably low
level;
· achieving a full understanding of the thorium
fuel cycle.
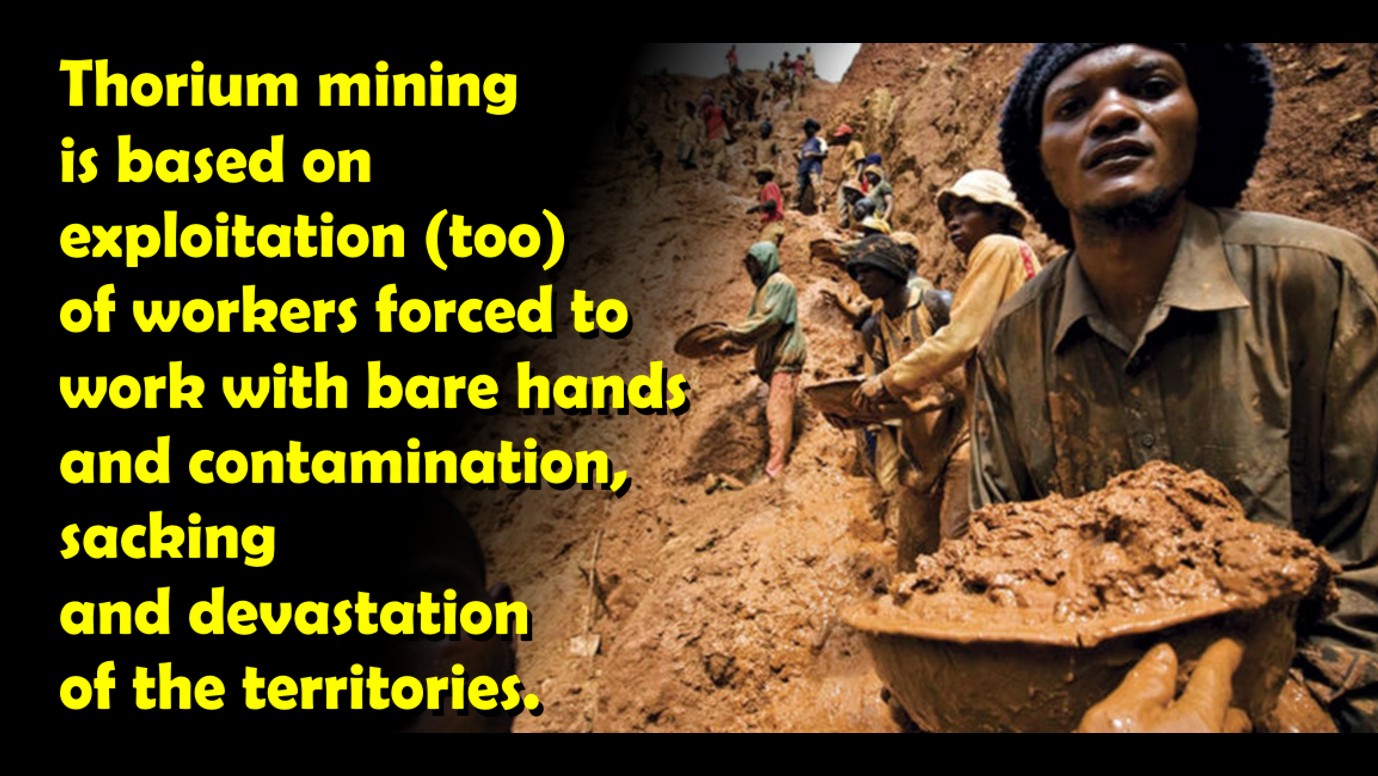
☣ Nuclear waste
Claim: LFTRs produce far less nuclear
waste than conventional solid fuel reactors.
Response: LFTRs are theoretically
capable of a high fuel burn-up rate, but while
this may indeed reduce the volume of waste, the
waste is more radioactive due to the higher
volume of radioactive fission products. The
continuous fuel reprocessing that is
characteristic of LFTRs will also produce
hazardous chemical and radioactive waste streams,
and releases to the environment will be
unavoidable.
Claim: Liquid fluoride thorium
reactors generate no high-level waste material.
Response: This claim, although
made in the report from the House of Lords, has
no basis in fact. High-level waste is an
unavoidable product of nuclear fission. Spent
fuel from any LFTR will be intensely radioactive
and constitute high level waste. The reactor
itself, at the end of its lifetime, will
constitute high level waste.
Claim: the waste from LFTRs contains
very few long-lived isotopes, in particular
transuranic actinides such as plutonium.
Response: the thorium fuel cycle
does indeed produce very low volumes of
plutonium and other long-lived actinides so long
as only thorium and 233U are used as fuel.
However, the waste contains many radioactive
fission products and will remain dangerous for
many hundreds of years. A particular hazard is
the production of 232U, with its highly
radio-toxic decay chain.
Claim: LFTRs can 'burn up' high level
waste from conventional nuclear reactors, and
stockpiles of plutonium.
Response: if LFTRs are used to 'burn
up' waste from conventional reactors, their fuel
now comprises 238U, 235U, 239Pu, 240Pu and other
actinides. Operated in this way, what is now a
mixed-fuel molten salt reactor will breed
plutonium (from 238U) and other long lived
actinides, perpetuating the plutonium cycle.
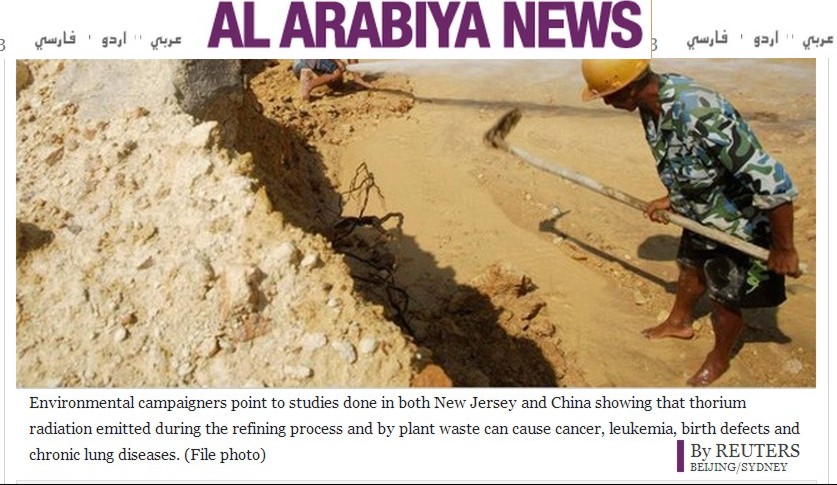
☣ Cost of electricity
Claim: the design of LFTRs tends
towards low construction cost and very cheap
electricity.
Response: while some elements of
LFTR design may cut costs compared to
conventional reactors, other elements will add
cost, notably the continuous fuel reprocessing
using high-temperature 'pyro-processing'
technologies. Moreover, a costly experimental
phase of ~20-40 years duration will be required
before any 'production' LFTR reactors can be
built.
It is very hard to predict the cost of the
technology that finally emerges, but the
economics of nuclear fuel reprocessing to date
suggests that the nuclear fuel produced from
breeder reactors is about 50 times more
expensive than ‘virgin’ fuel. It therefore
appears probable that any electricity produced
from LFTRs will be expensive.
We must also consider the prospect that
relatively novel or immature energy sources,
such as photovoltaic electricity and
photo-evolved hydrogen, will have become well
established as low-cost technologies long before
LFTRs are in the market.
☣ Timescale
Claim: Thorium and the LFTR offer a
solution to current and medium-term energy
supply deficits.
Response: The thorium fuel cycle
is immature. Estimates from the UK’s National
Nuclear Laboratory and the Chinese Academy of
Sciences (see 4.2 below) suggest that 10-15
years of research will be needed before thorium
fuels are ready to be deployed in existing
reactor designs. Production LFTRs will not be
deployable on any significant scale for 40-70
years.
La "intro" è a cura della Redazione di RNA